An international team of researchers including a team from the Center for the Advancement of Topological Semimetals (CATS), an Energy Frontier Research Center under the U.S. Department of Energy’s Office of Science led by Ames National Laboratory, experimentally demonstrated a new type of nonlinear Hall effect. This Hall effect is driven by the quantum metric, which defines the distances between electronic wavefunctions inside a crystal. The experimental work was led by scientists at Harvard University while the theoretical modeling was developed at Ames Lab. This project provides the first experimental proof of the nonlinear Hall effect, which has only been theorized to this point.
Peter Orth, who led theory efforts at Ames as part of the CATS project, explained that the theoretical modeling was necessary to understand what was happening during the experiment. Orth and postdoctoral scholar Thais V. Trevisan utilized their expertise to unravel the complex interactions revealed by the experiments.
The material used for this research consists of manganese-bismuth-tellurium (MnBi2Te4 or MBT). To see the phenomenon, Orth explained that the specimen must have a special symmetry. Orth said, “But if you just take pure MBT, this phenomenon is actually zero by symmetry.” So, the experimentalists added layers of black phosphorus (BP), which has a different symmetry, on the top and bottom of the MBT. The BP interacts with the MBT, breaking the symmetry and resulting in a nonzero nonlinear Hall response.
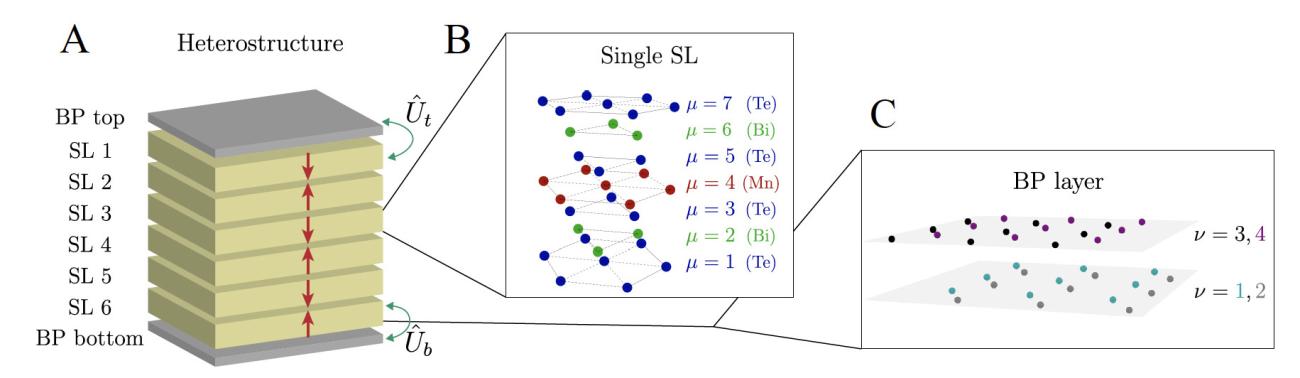
According to Orth, the goal of this experiment was to understand the origin of the quantum metric nonlinear Hall effect. They wanted to understand if it is driven by lattice strain or by electronic mixing between MBT and BP layers.
“Our contribution was to theoretically model this system. This is pretty challenging because we have these two materials, MBT and BP which have different lattice constants and different symmetries”, Orth said. “It's like an engineering challenge.”
“When you interface two systems, you have basically one lattice imposing strain on the other system and reducing the symmetry. That's like an effect where you shift the atoms around,” Orth explained. “Or you could have electronic coupling, so the electrons hop from one lattice layer to the other part of the system and hop back, and it would be more like a mixing of the electronic states.”
Incorporating both the lattice strain and electronic mixing into the model made it even more complex, “But this electronic mixing is very important in order to capture these experimentally observed effects,” Orth said. The calculations revealed that the phenomenon comes from both the strain between the two different lattices and electronic mixing with different experimental features associated with the two mechanisms.
The team was successful in their proving that the experimental results originate from the quantum metric nonlinear Hall Effect. CATS Director and Iowa State University Professor Rob McQueeney commented that “The results are at the heart of what we are trying to achieve in CATS. New materials with exciting and predictable responses such as the quantum metric Hall effect await discovery and may ultimately serve as future platforms for electronic components or sensors.”
This research is further discussed in “Quantum metric nonlinear Hall effect in a topological antiferromagnetic heterostructure,” written by Anyuan Gao, Yu-Fei Liu, Jian-Xiang Qiu, Barun Ghosh, Thaís V. Trevisan, Yugo Onishi, Chaowei Hu, Tiema Qian, Hung-Ju Tien, Shao-Wen Chen, Mengqi Huang, Damien Bérubé, Houchen Li, Christian Tzschaschel, Thao Dinh, Zhe Sun, Sheng-Chin Ho, Shang-Wei Lien, Bahadur Singh, Kenji Watanabe, Takashi Taniguchi, David C. Bell, Hsin Lin, Tay-Rong Chang, Chunhi Rita Du, Arun Bansil, Liang Fu, Ni Ni, Peter P. Orth, Qiong Ma, and Su-Yang Xu, and published in Science.
Work for this research was partly supported by the Center for the Advancement of Topological Semimetals (CATS), an Energy Frontier Research Center funded by the U.S. DOE, Office of Basic Energy Sciences.
Ames National Laboratory is a U.S. Department of Energy Office of Science National Laboratory operated by Iowa State University. Ames Laboratory creates innovative materials, technologies, and energy solutions. We use our expertise, unique capabilities, and interdisciplinary collaborations to solve global problems.
Ames Laboratory is supported by the Office of Science of the U.S. Department of Energy. The Office of Science is the single largest supporter of basic research in the physical sciences in the United States and is working to address some of the most pressing challenges of our time. For more information, please visit https://energy.gov/science.