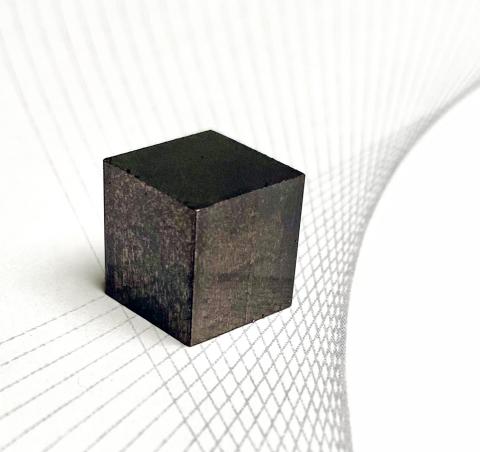
Many of the industrial motors used today are induction motors. However, permanent magnet motors are generally more efficient than induction motors and can be more cost-effective in the long run, especially for factories and plants that run constantly. Currently, most permanent magnet motors rely on critical materials, specifically rare-earth based high-performance magnets, which are costly and exceed the performance needs of many industrial applications.
Scientists are working to find alternatives to rare-earth-based magnets that can function well in motors, because rare earth materials are expensive and can experience supply chain issues. However, finding a cost-effective, functional alternative material is challenging. A motor magnet needs strong magnetism that is unaffected by high temperatures and exposure to magnetic interference.
Magnets have this property called coercivity. Coercivity is the measure of the magnet’s ability to resist demagnetization when it is exposed to an external magnetic field. High temperatures can cause magnets to lose their coercivity. Industrial motors run at very high temperatures, so a permanent magnet designed for an industrial motor needs to maintain its coercivity at very high temperatures.
One solution to this challenge was discovered by a team of researchers and industry partners led by scientists at the U.S. Department of Energy Ames National Laboratory. They developed a rare-earth-free bonded magnet that not only retains its magnetism at high temperatures, coercivity nearly doubles with a 100°C (212°F) increase from room temperature.
“You cannot use rare earths to solve everything. That's an awful waste of a critical natural resource,” said Jun Cui, a scientist at Ames Lab and the team leader.
The magnet the team developed is a combination of manganese and bismuth (MnBi). The key to its performance lies in the preparation and fabrication process.
Magnets have these tiny crystal-like structures called grains that appear at the microscopic level and can influence magnetic behavior. Cui explained that the interaction between these grains can have a negative impact on magnetic performance.
“We don’t want those grains to touch each other,” explained Cui. “If they touch, they will establish some sort of a communication, and it takes only one imperfect grain to convince the whole neighborhood and create an avalanche-loss of magnetism.”
To prevent grain interactions, Cui’s team developed a process to isolate the grains from each other during the fabrication process. They start by turning the material into an extremely fine powder. Next, they coat each of the particles with a delicate polymer solution that prevents grain-to-grain contact.
During fabrication, the researchers use an external magnetic field to align the particles. This process results in formation of an anisotropic magnet, explained Wei Tang, also a scientist at Ames Lab. Anisotropic magnets have a preferred direction of magnetization, which enhances the magnet’s overall performance.
Cui also pointed out that bismuth, one of the key elements in this new magnet, is not only naturally available but is also a byproduct of smelting and refining other materials. Utilizing the byproduct contributes to cost saving and resource efficiency for this material, making it an economical choice.
Cui and Tang explained that they did have to sacrifice some magnetic force when crafting this magnet to have high coercivity, but this tradeoff aligns with the specific needs of many industrial applications.
“If we look at the whole world right now, actually, motors can operate without magnets,” said Cui. “But on the other side, high efficiency motors require high-performance magnets. We are developing something in between, and offering a balanced solution: an affordable, non-rare-earth magnet for targeted industrial tasks.”
The team has already collaborated with an industrial partner to test this magnet in an industrial pump motor. The motor performed slightly better than the design specifications. The industry partners have already moved on to “fatigue tests,” where they assess the performance and endurance of the new magnet motor.
“I am excited because we’re doing lots of research, and some research just gets published in a paper. But this magnet is non-rare earth, which is very significant,” said Tang. “And we are very close to achieving real-world applications for this magnet. If successful, this would be the first in the world for industrial application of the non-rare-earth MnBi magnet.”
“As a scientist, the ultimate reward is seeing your work make a real-world impact,” said Cui.
Ames National Laboratory is a U.S. Department of Energy Office of Science National Laboratory operated by Iowa State University. Ames Laboratory creates innovative materials, technologies, and energy solutions. We use our expertise, unique capabilities, and interdisciplinary collaborations to solve global problems.
Ames National Laboratory is supported by the Office of Science of the U.S. Department of Energy. The Office of Science is the single largest supporter of basic research in the physical sciences in the United States and is working to address some of the most pressing challenges of our time. For more information, please visit https://energy.gov/science.