The Division of Materials Sciences and Engineering (DMSE) performs materials research across a broad spectrum. We confront grand science challenges, conducting discovery research which addresses fundamental limitations in our understanding of complex states of matter. Our directed research guides the design of new materials to applications in advanced energy technologies.
Basic research conducted within the DMSE is performed primarily through funding provided by the U.S. Department of Energy’s Office of Basic Energy Sciences. Our directed research also receives funding from DOE technologies offices including the Office of Energy Efficiency and Renewable Energy and the Office of Fossil Energy as well as strategic partnership projects.
News & Highlights
Ames National Laboratory scientist and inventor Iver Anderson retires

Researchers address material challenges to make commercial fusion power a reality

The future of more sustainable cooling and heating technology could be just around the corner
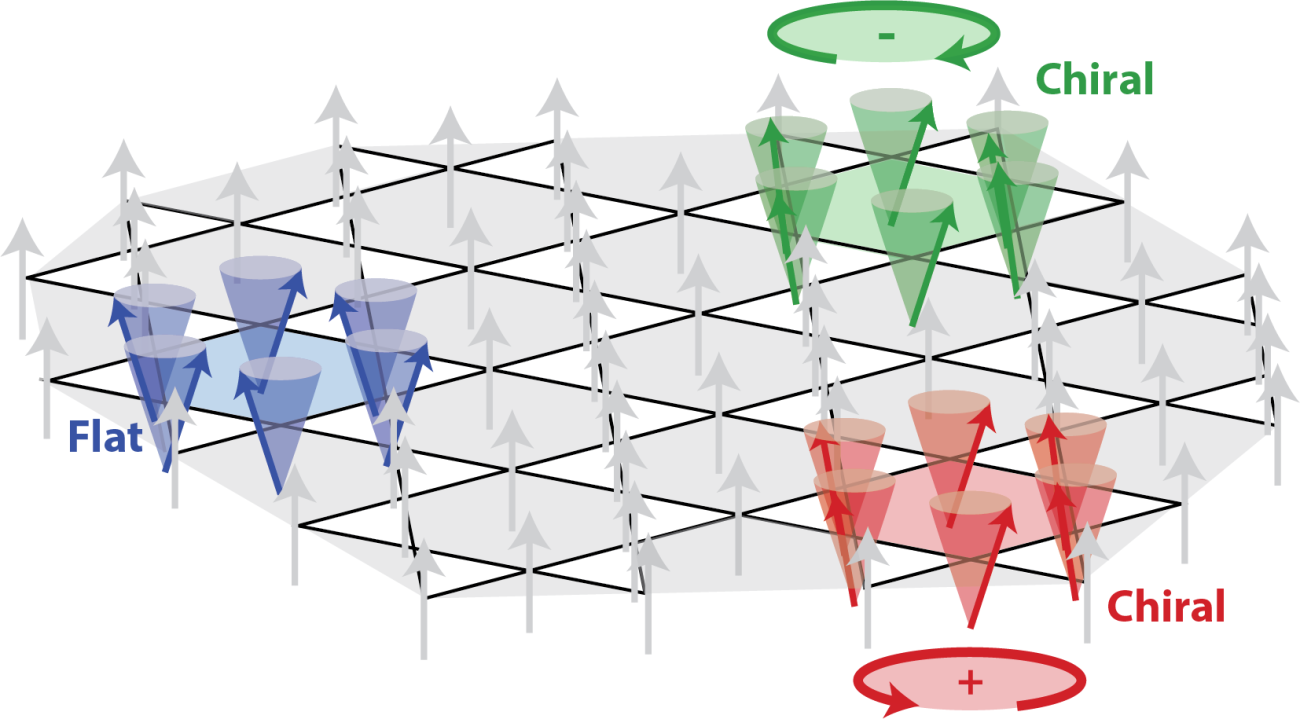
Researchers find unexpected excitations in a Kagome layered material
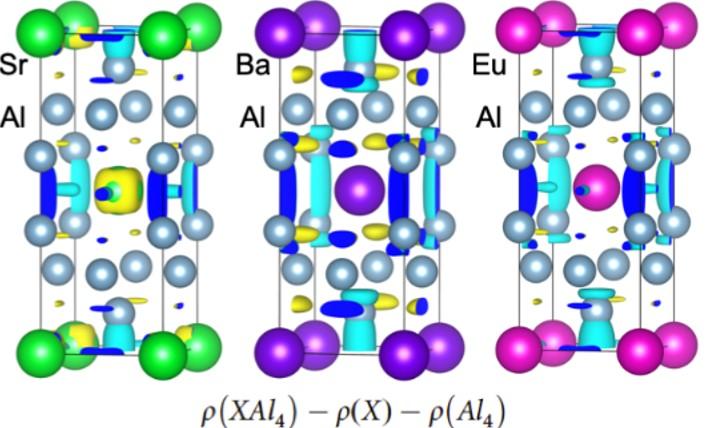